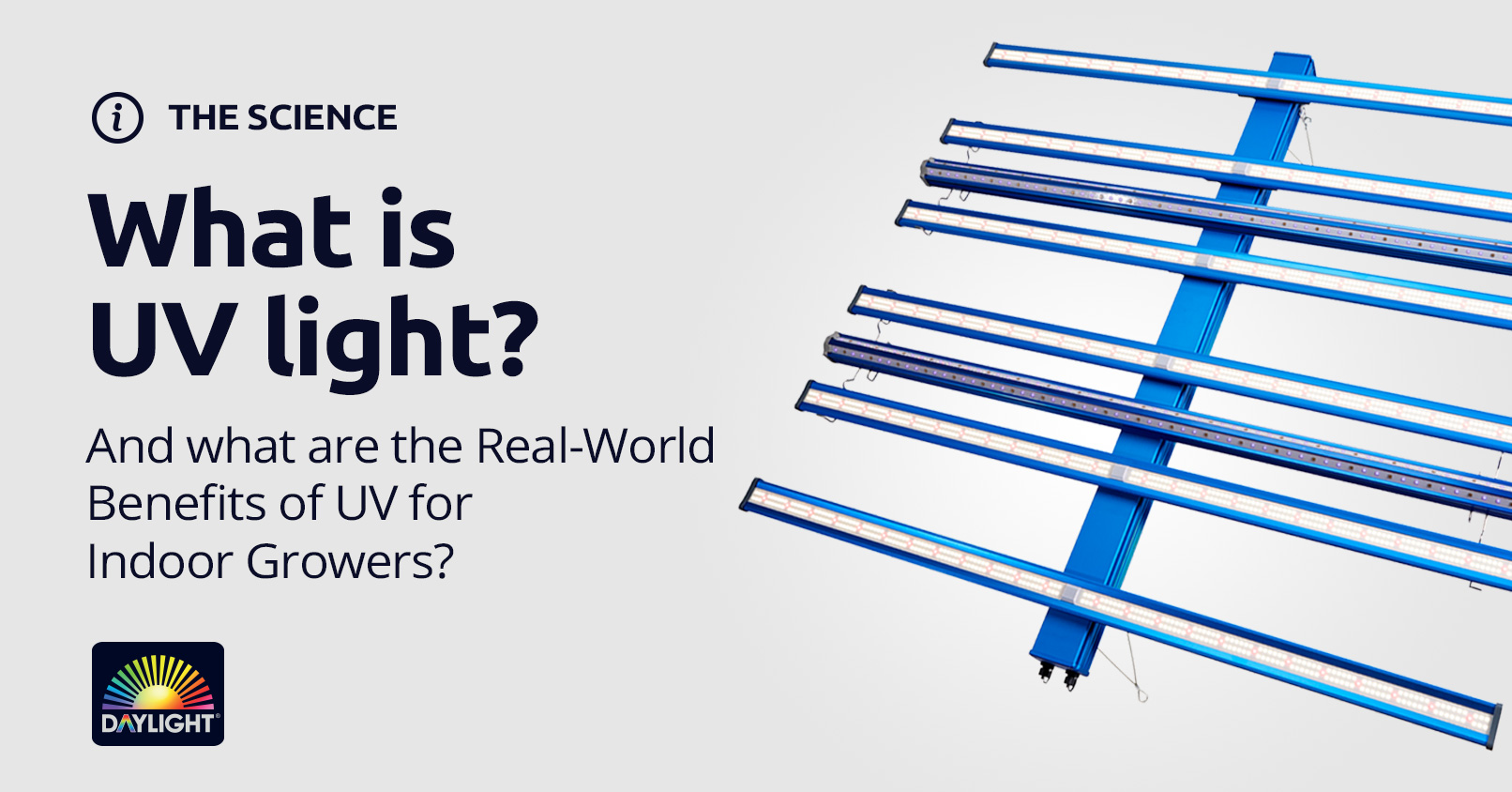
What are the Real-World Benefits of Ultraviolet (UV-A, UV-B, UV-C) for Indoor Growers?
There has been persistent chatter about ultraviolet (UV) radiation among indoor growers for decades. We know that around ten percent of solar radiation is UV—with about three quarters of it absorbed by the atmosphere. At ground level at midday, incident solar radiation is made up of 44% PAR / visible light, 3% ultraviolet, and the remainder, around 53%, is infrared.
Incident UV radiation (the UV which reaches the surface of our planet) increases with altitude due to the thinning of the atmosphere. The same is also true of near infrared.
Now, before we dive deeper into UV, we need to understand that it is a wide category of electromagnetic radiation—at least, wider than PAR (photosynthetically active radiation)—and so it’s useful to break it up into subdivisions. The visible spectrum of colours is defined as photons traveling at wavelengths between 400 and 700 nanometres (nm), from violet (400 nm) to red (700 nm). As its name suggests, UV is radiation beyond violet—i.e. its wavelengths are less than 400 nm. However, UV extends all the way down to wavelengths of just 10 nm. Beyond that, electromagnetic radiation is classified as X-rays and gamma rays!
Safety First!
When working with any bright artificial light source, it’s always a good idea to wear eye protection in the form of quality sunglasses. If your light source contains high levels of UV then it is best to avoid working within the grow room whilst the UV light is switched on.
For the purposes of this discussion, we’re going to divide UV in four subtypes: Extreme UV, UV-C, UV-B and UV-A. Extreme UV describes photons with wavelengths between 10 and 121 nm. This is the highest energy UV and the most dangerous. It is classified as “ionizing radiation” meaning it has the power to break molecular bonds. UV-C (100 – 280 nm) also has the power to ionize, especially at its more energetic, lower wavelengths. Exposure to extreme UV and UV-C can cause skin cancer but artificial forms of UV-C are useful for sterilization / germicidal applications. Fortunately for all of us, virtually all extreme UV and UV-C produced by the Sun is absorbed by our planet’s ozone layer before it has a chance to fry us.
The two forms of UV that are of most interest and relevance to both humans and plants are UV-B (280 – 315 nm) and UV-A (315 – 400 nm). Of the relatively small fraction of UV that reaches our planet, the vast majority (around 95%) is the more benign UV-A and 5% UV-B. Most of the UV-A we receive is closer to visible light than UV-B.
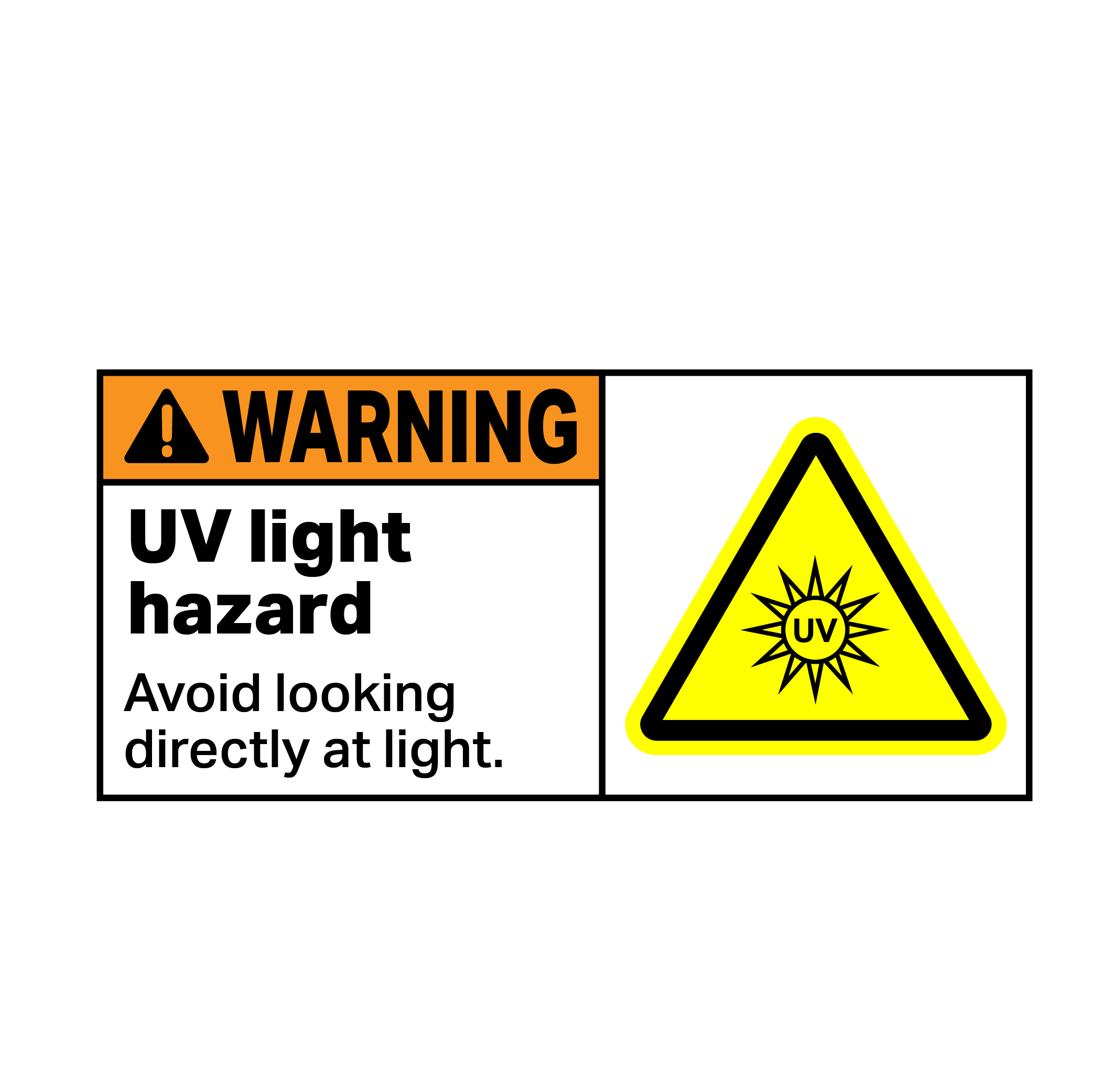
Benefits of UV-A in Plant Lighting
- Increase photosynthetic rates when supplied in conjunction with PAR light
- Stronger, more robust seedlings / cuttings in propagation
- Offset the stretching effect of far-red
- Help ward off pests
- More accurately recreate lighting diet of higher altitude species
- Stimulate more, flavonoids, stilbenes and other beneficial compounds
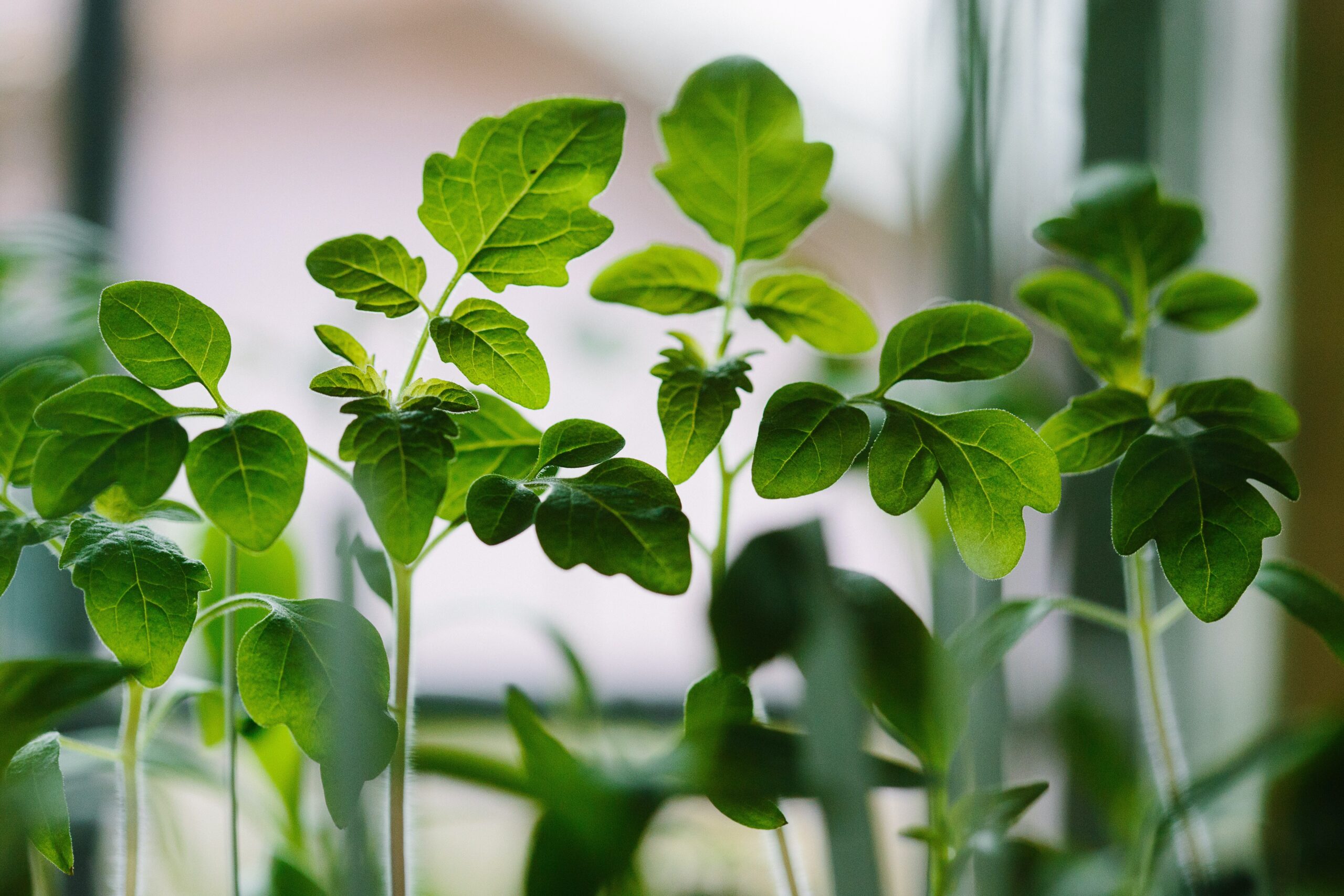
UV-A
UV-A, as well as blue light, can both help to counteract the stretching effect of red and far-red light as well as promote photoprotective responses such as the production of UV and blue light absorbing pigments. UV-A can also contribute to photosynthesis when delivered in conjunction with full spectrum light and also help to increase the thickness of leaves, making them less vulnerable to pathogenic fungal species and pests. There is a strong argument, therefore, for incorporating a small amount of UV-A into the lighting regimen for seedlings, cuttings and young plants. Too much of a good thing can also be bad—and this is certainly true of UV-A. Growers using discrete UV-A light sources should do so sparingly, timing the UV to operate for a few hours during the middle of the photoperiod.
An excess of UV can cause DNA damage to plants (and humans) but some plant species produce an enzyme called photolyase which helps to repair damaged DNA. Plant species native to higher altitudes have enhanced photolyase production and some plant species even go as far as producing their own “sun screen” in the form of essential oils.
UV-B
UV-B can increase the production of flavonoids and essential oils in many adapted plant species. It is essential that UV-B is delivered in tandem with high levels of PAR light in order to mitigate the cellular damage caused by UV-B alone. Some plant species have the gene UVR8 which helps to mitigate UV-B damage by stimulating the production of photoprotective substances. UVR8 is most sensitive at 285 nm, towards the lower limit of UV-B.
Remember, only 5% of the small amount of UV that reaches our planet is UV-B but UV-B photons pack an incredibly powerful punch—it should therefore be used with caution and moderation.
Benefits of UV-B in Plant Lighting
- Increase photosynthetic rates when supplied in conjunction with PAR light
- Strengthens plant so that it can cope with and exploit higher light intensity
- Stimulate the UVR8 plant gene which can trigger essential oil production and boost crop quality
- Offset the stretching effect of far-red
- Thickens leaf tissue and waxiness—making it harder for pests and pathogenic fungi to get a foothold
- Sterilises water-borne bacteria and associated mould spores
- More accurately recreate lighting diet of higher altitude species
- Stimulate more, flavonoids, stilbenes and other beneficial compounds
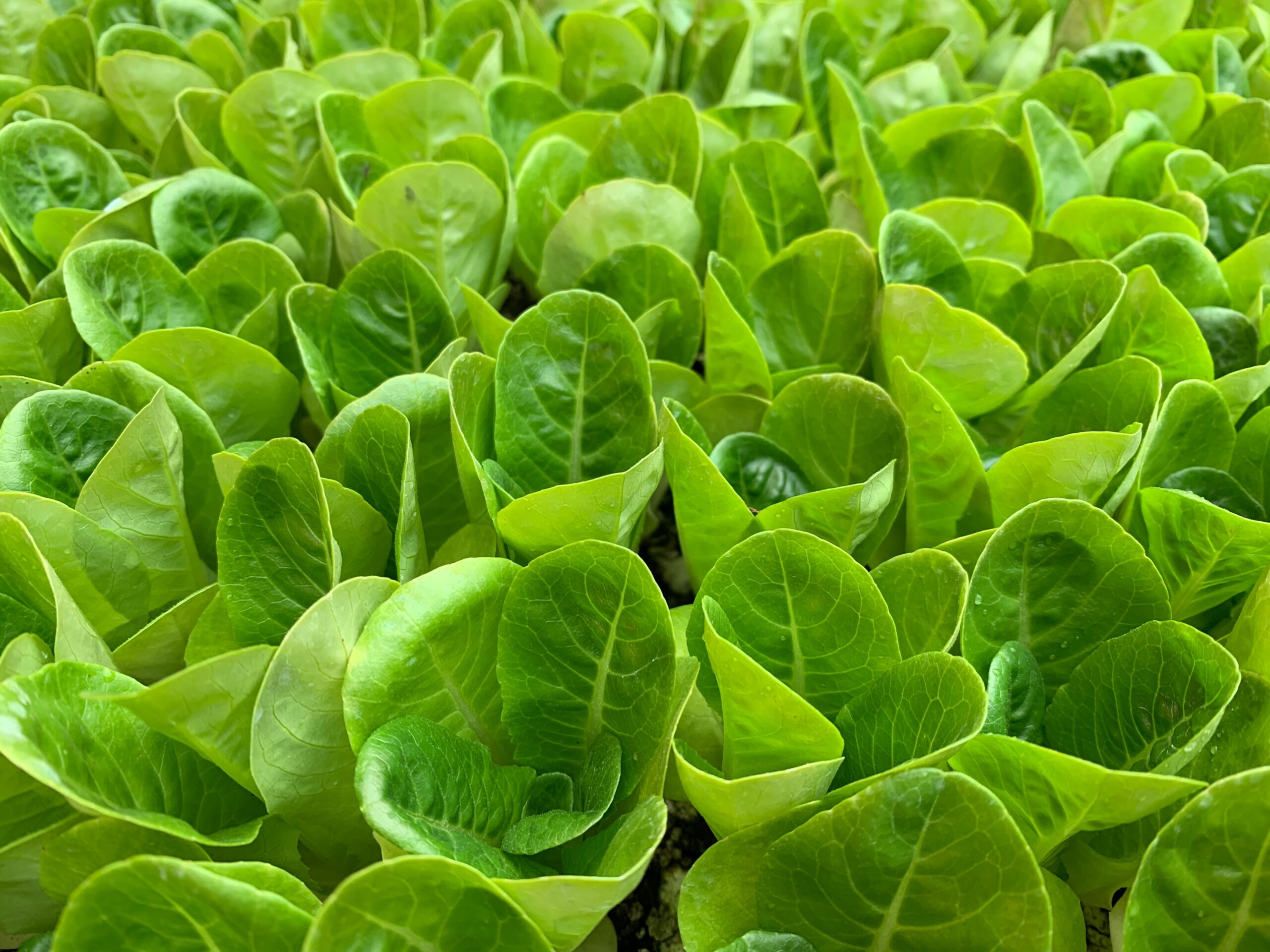
UV and Grow Lights
Okay, so perhaps you’re convinced that you want to add some UV to your plants’ spectral diet. This naturally raises the question: how? Let’s run through the different grow light technologies and see how they compare:
T5 Fluorescent
Full spectrum T5 lamps are now available with additional UV-A. Dedicated UV-A and UV-B T5 lamps are also available.
Light Emitting Plasma (LEP)
LEP produces a continuous full spectrum with some UV-B and UV-A.
Metal Halide (MH)
Metal halide lamps produce a useful amount of UV-A (starting at around 350 nm)
CMH / DE-CMH
4K ceramic metal halide lamps are arguably the most reliable and cost-effective source of UV-B and UV-A. Look for horticultural grade lamps with no UV blockers (CMH designed for retail environments have UV blockers.)
HPS / DE-HPS
No UV is produced from HPS / DE-HPS.
LEDs
Reliable and efficient UV-A diodes are available which output peak wavelengths of 395 nm, 380 nm and 360 nm. UV-B diodes are much more expensive and less efficient.
Conclusion
As more reliable forms of artificial UV emerge along with our expanded understanding of UV’s role in plant morphology and photosynthesis, quality-focused indoor growers are moving away from “pure PAR” and embracing fixtures which incorporate some UV into their spectral mix. Even though this strategy inevitably reduces PPF and PPE numbers, growers are learning that it’s not all about blasting their plants with high PPFD. As light intensity increases, spectral quality becomes more important—and UV clearly has a major role to play.
Further reading
Brazaitytė, A., et al. “Effect of supplemental UV-A irradiation in solid-state lighting on the growth and phytochemical content of microgreens.” International Agrophysics 29.1 (2015): 13-22.
“Almost all supplemental UV-A irradiation treatments resulted in increased leaf area and fresh weight.”
Mantha, Sailaja V., Gregory A. Johnson, and Thomas A. Day. “Evidence from Action and Fluorescence Spectra that UV‐Induced Violet–Blue–Green Fluorescence Enhances Leaf Photosynthesis.” Photochemistry and photobiology 73.3 (2001): 249-256.
“340 nm UV-A enhanced photosynethesis by 8-10% with non-saturating background PAR at 500 umol m-2s-1.”
Turnbull, Tarryn L., Alexandra M. Barlow, and Mark A. Adams. “Photosynthetic benefits of ultraviolet-A to Pimelea ligustrina, a woody shrub of sub-alpine Australia.” Oecologia 173.2 (2013): 375-385.
“Increased photosynthesis by 12%.”
Chang, Chung-Liang, and Kuang-Pi Chang. “The growth response of leaf lettuce at different stages to multiple wavelength-band light-emitting diode lighting.” Scientia Horticulturae 179 (2014): 78-84.
“Increased leaf size and dry weight of plant.”
Wang, G., M. Gu, J. Cui, K. Shi, Y. Zhou, and J. Yu. 2009. Effects of light quality on CO2 assimilation, chlorophyllfluorescence quenching, expression of Calvin cycle genes and carbohydrate accumulation in Cucumis sativus. J. Photochem. Photobiol., B 96:30–37.
“Cucumber plants grown under UVA light were found to have higher photosynthetic potential and increased transcription of the genes required for carbon fixation compared to plants grown under red, green, or yellow light”