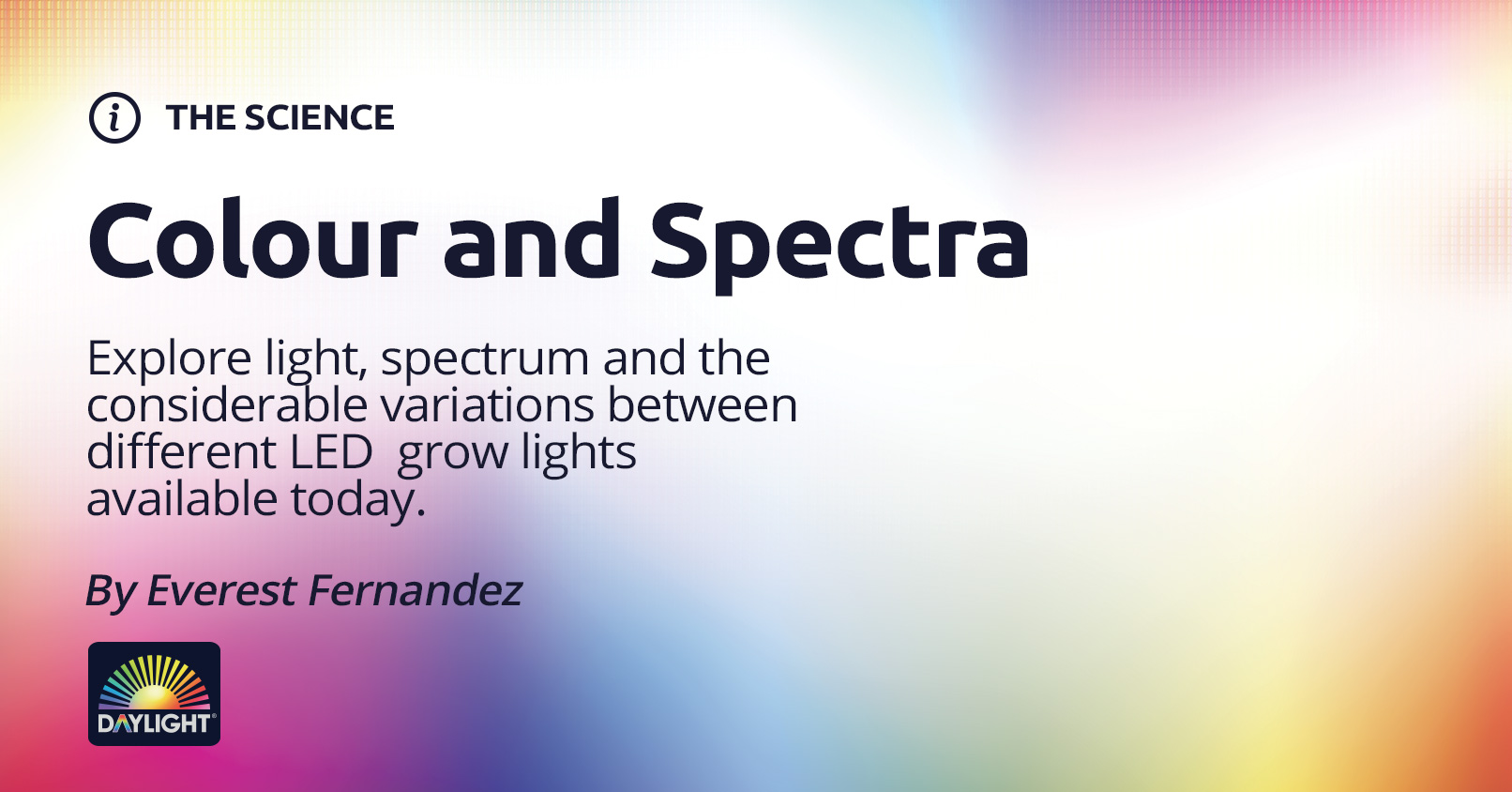
The Goal
High performance, LED grow lights all share a common, primary job: to provide the light required by plants (typically light-loving / high DLI species) to grow, bloom and fruit efficiently and productively. Why is it then that the light spectra generated by one LED grow light can differ so markedly from that of another? Are some lights optimized for certain plant species, life-cycle stages, or specific environmental conditions? Why do some LED grow light manufacturers offer their fixtures with different spectral options, or even the ability to “dial in” different light recipes?
The Diodes
To approach these potentially tricky questions, we need to delve into the diodes themselves and how light itself is utilized by plants. The light produced by LED grow light fixtures is aggregate output from hundreds, sometimes thousands, of tiny diodes. Different types, or colours, of diode are arrayed together in various combinations and patterns to create a given overall spectrum. Monochromatic diodes emit narrow bandwidths of light, e.g. 395 nm UV-A diodes, 420 nm blue, 660 nm red, and 730 nm far-red. The output of these “mono” diodes typically only spans around 15 nm. Other diodes are known as “broad spectrum,” “full spectrum,” or more plainly as “white light”. These diodes are housed within a phosphor coating which transforms the output of blue monochromatic diodes into broader spectrum light, spanning the entire visible spectrum. Different flavours of full spectrum diode are available, ranging from warm yellowish whites (typically rated around 3000 Kelvin) to cool, blueish whites (5000 Kelvin and higher).
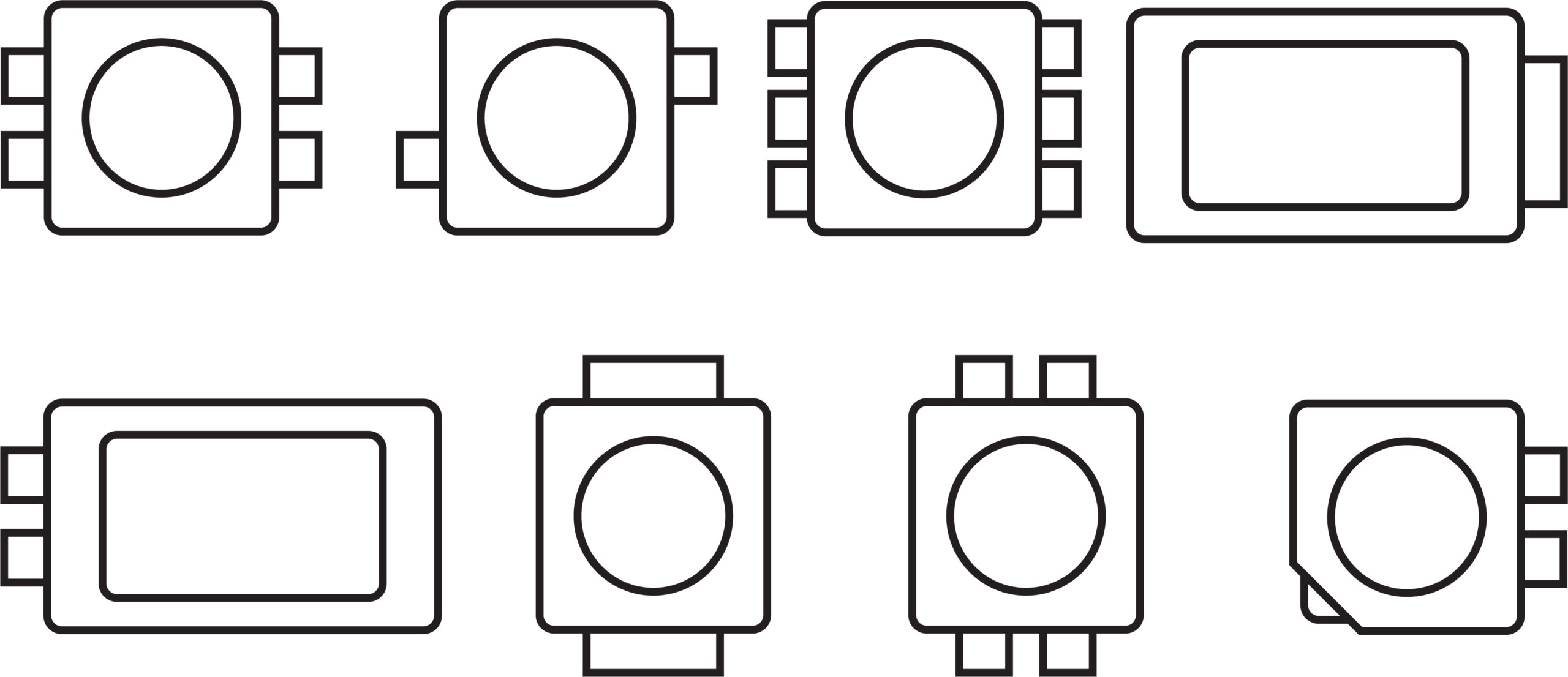
The Fixtures
These days, most LED grow lights on the market are comprised mainly of full spectrum diodes (3000 Kelvin or 5000 Kelvin, or both), supplemented with high-efficiency, monochromatic reds. Some run fewer diodes at higher currents, whereas others opt for higher diode numbers on lower currents. You will often see mono blue diodes (420, 435 nm) and UV-A (380, 395 nm), some add far-red (730 nm). The resulting marketplace can be somewhat confusing, with grow lights offering quite a range of different spectra, even among those purportedly designed for indoor use with light-loving plant species.
The Plants
Let’s turn our attention now to how plants interact with light and see if we can reveal any clues about what type, or spectrum, of light is best. Plants contain a variety of special materials called pigments. Biological pigments are naturally-occurring substances that give colour to living tissue and, unsurprisingly, are found pretty much everywhere! Just look in the mirror—the irises of your eyes are a certain colour (or colours) because they contain a characteristic mix of pigments. Similarly, your skin and hair, the stems, leaves, flowers and fruits of plants, as well as the feathers and fur of animals, all contain pigments.
While we can all agree that it’s awesome to live in a glorious, technicolour world, pigments do a lot more than help to beautify our planet. Some plant pigments are responsible for photosynthesis—the conversion of carbon dioxide and water into energy stores called carbohydrates. This process is driven by the energy contained in light. The cosmic source of this energy is the nuclear fusion of four hydrogen atoms into helium, mostly taking place in the core of the Sun. The ultimate conversion of this energy into carbohydrates is the battery that drives all plant metabolic processes.
Chlorophyll
Before we delve deeper into qualities of plant-usable light, we should note that, in contrast to other types of colourful natural phenomena, we perceive pigments as “colours” because of their special ability to selectively absorb certain wavelengths of visible light. The colours we see are the result of reduction of these absorbed wavelengths from full spectrum “white” light. The reason we see healthy plant leaves as “green,” for example, is because healthy foliage is abundant in a pigment called “chlorophyll”—specifically, chlorophyll A and B, both of which absorb red and blue light very effectively. As sunlight (or light from a ‘white’ or broad spectrum artificial light source) is made up of all the visible colours, from violet to red, we perceive healthy foliage as “green” because it’s the portion of the visible light spectrum that’s not so efficiently absorbed.
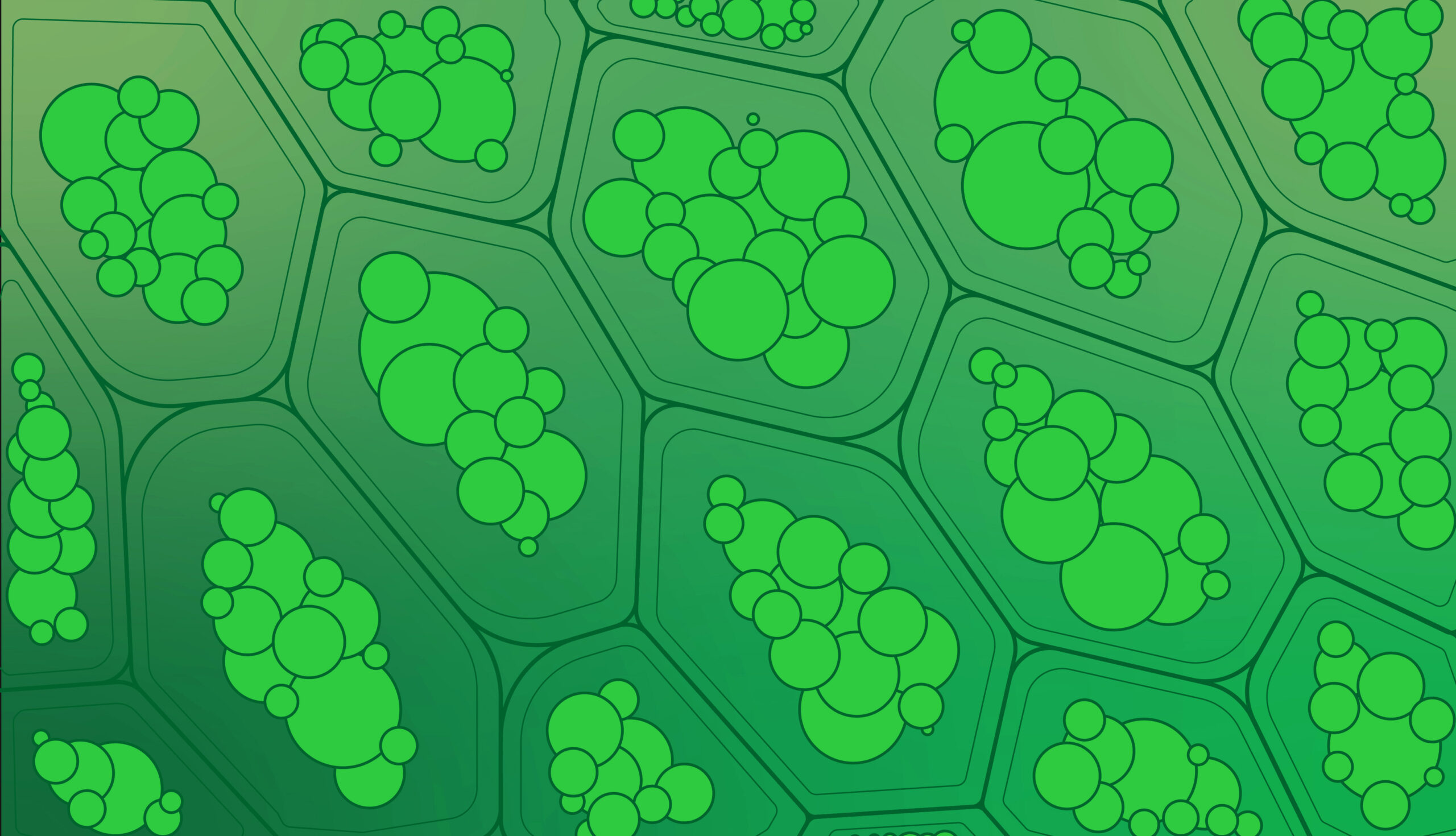
Other Pigments
While chlorophylls are arguably the most well-known of the plant pigments, there’s a lot more to the story than that. Plant pigments can be divided into three major categories: chlorophylls, carotenoids and flavonoids. Different plant species contain different mixes of pigments, a result of millions of years of evolution and adaptation to various environments. Variation can even be seen between different cultivars of the same plant species. Note that the photons absorbed by carotenoids can be transferred to chlorophyll.
Chlorophylls are the primary drivers of photosynthesis, but carotenoids also provide essential assistance. They can pass electrons (excited by incoming light) to chlorophyll A as well as offering protection against excessively intense light. These “harder wearing” pigments are responsible for the yellow and orange colorations we associate with Autumn leaves, but they are present in healthy foliage throughout the lifecycle. (Their colours are masked by chlorophyll.) Carotenoids only reveal themselves as the surrounding chlorophyl begins to degrade at the end of the growing season.
The third group of plant pigments are known as flavonoids, which are found in the vacuoles of cells. The most common flavonoids are called “anthocyanins” which produce a bright red coloration. Flavonoids help to protect the photosynthetic apparatus of plants against photoinhibition under excessive light intensity and absorb ultraviolet radiation. There are other plant pigments known as phytochromes and, while this class of pigments also absorbs red and far-red light, phytochromes act more like a switch than an energy converter, helping plants to regulate processes such as seed germination, stem elongation, or the transition from vegetative growth to generative development (flowering and fruiting).
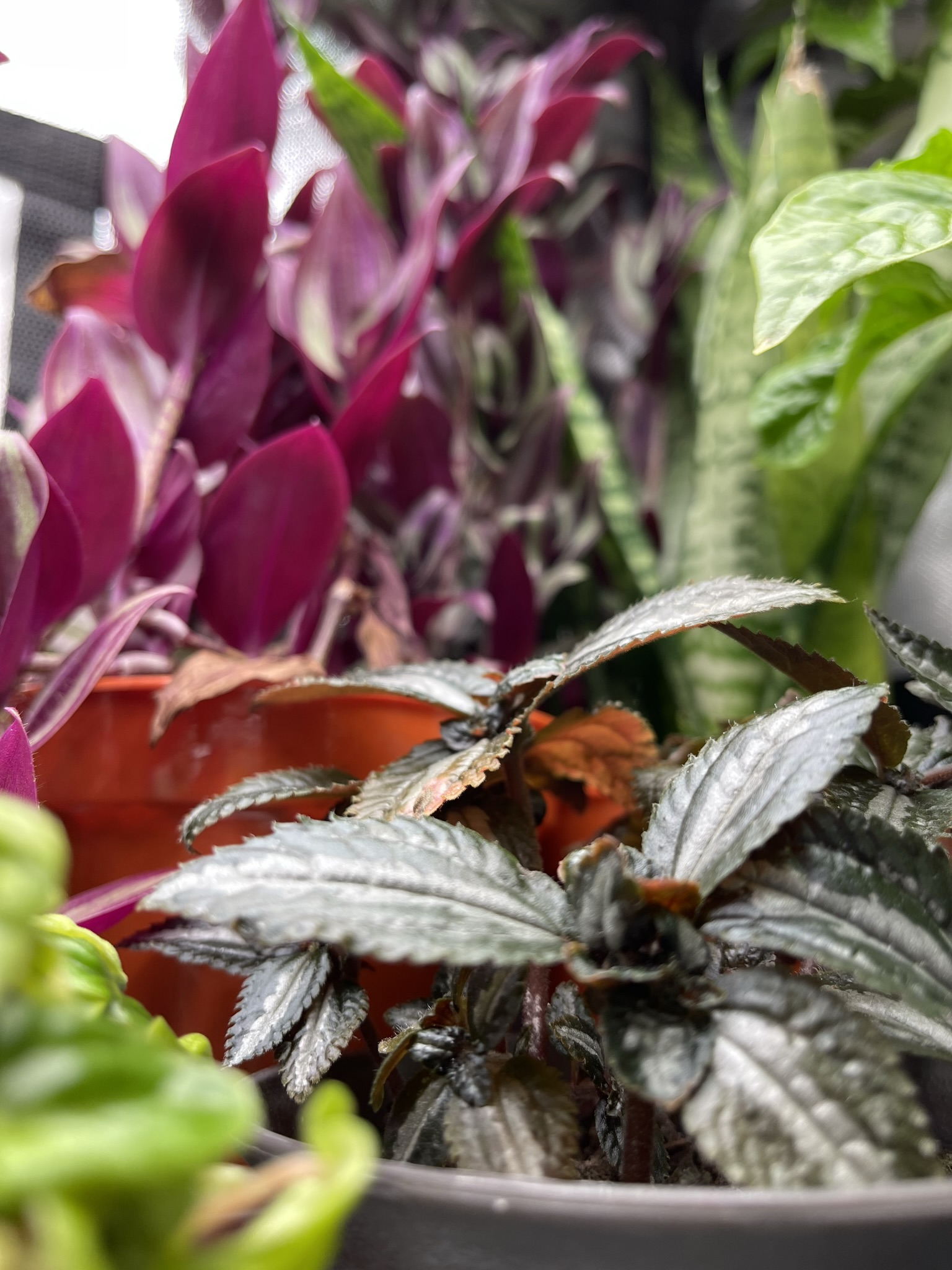
Towards an Optimal Spectrum for Flowering/Fruiting Plants
Chlorophyll A is a blueish green colour and absorbs light most efficiently traveling at 420 and 660 nanometres. Chlorophyll B is more yellowish-green and absorbs light most efficiently at 435 and 643 nanometres. However, there is typically only a third of chlorophyll B present in chloroplasts compared with chlorophyll A.
The key carotenoids in plants are β-carotene (425, 450 and 480 nm), α-carotene (420, 440 and 480 nm) and Luteol (425, 445 and 485 nm).
It is now generally accepted that eight or nine photons are required to liberate one molecule of oxygen in photosynthesis. Red light is the most energy-efficient form of plant-usable light. It takes less energy to produce a photon of red light than a photon of blue light, but plants photosynthesise more efficiently when both are present. Similarly, in the presence of red light, plants can also photosynthesise far-red light, whereas far-red light has little photosynthetic effect when supplied in isolation. Red light is also known to support flower and fruit production, as well as stem elongation.
The wavelengths considered to be most important for photosynthesis reside in the red (600−700 nm) and blue (420−450 nm) zones of the spectrum (the maximum absorption ranges for chlorophylls a and b, with carotenoids adding absorption in the 420−500 nm range). While early LED fixtures focused entirely on red and blue light, more recent studies have revealed that full spectrum (white light—or red and blue light with yellow and green “filler”) produce more plant growth and better-quality produce. Blue and green light is thought to assist with stomatal opening on the underside of leaves. Green light has recently been shown to stimulate photosynthesis deep within leaf tissue. The threshold for photoinhibition and phototoxicity appears to be higher for plants grown under broad spectrum light compared with light that is overly dominant in red or blue.
The Red:Blue Ratio
There are many ways to qualify light and spectrum, but perhaps the most pertinent for indoor growers is the red:blue ratio. It’s difficult to pinpoint an ideal ratio as the optimal requirements for different species and cultivars vary considerably. However, an efficient flowering spectrum will typically have a red:blue ratio in the region of 4:1. I.e. around four red photons for every blue (HPS lamps offer around a 6:1 ratio which is why growers historically paired them with adjacent metal halide fixtures to increase the blue.) Grow lights with higher blue content than red are better suited to vegetative growth. They will typically produce more compact plants with larger leaves. Blue light can certainly help to promote the production of secondary metabolites, adding to crop quality, but it should always come second to red for flowering and fruiting applications.
Summary
While spectra vary considerably between different LED grow light fixtures, red light is the most energy-efficient plant-usable light that we can produce. Light-loving, flowering and fruiting plants are most productive indoors when grown under a slightly red-dominant full spectrum. Red light can be supplied via red mono-diodes and full spectrum diodes. Plants can tolerate higher overall light intensity if the spectrum is balanced and broad because different primary and accessory pigments in the plant are stimulated simultaneously. While the optimal spectrum varies between different plant species (and even between different cultivars of the same species), the ideal ratio of red photons to blue (to drive flowering and fruiting) is around 4:1.
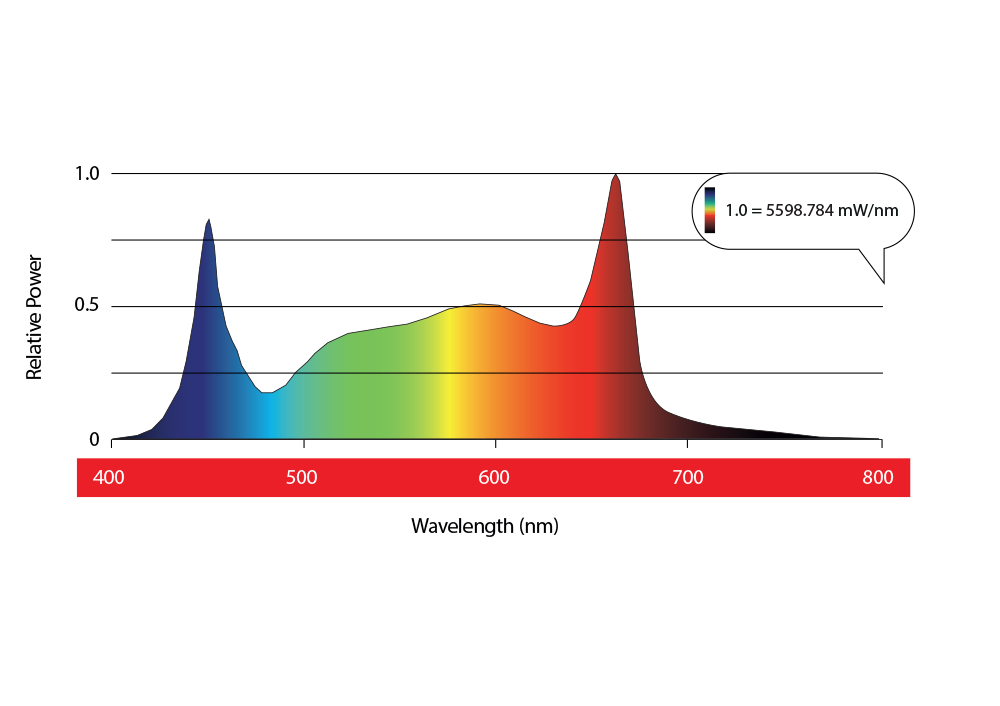